Fields And Particles.
1. Are Quantum Fields Real? (See Full Article Below.)
2. How Do Quantum Fields Create Particles? (See Full Article Below.)
3. When Did The Universe Get Its First Quantum Fields? (See Full Article Below.)
4. What Is A Force? Revisited. (See My Quick Take Below.)
Traditional Fields Viewpoint. Everyday Forces.
Versus Force-Carrier Particles Viewpoint. In Subatomic Particle Interactions.
Maths Secured? And Also, The Above Prerequisite Article Series Before This Book Read:

Ask Ethan: Are Quantum Fields Real?
Ethan Siegel Senior Contributor Starts With A Bang Contributor Group Science The Universe is out there, waiting for you to discover it.
Nov 17, 2018,10:00am EST
https://www.forbes.com/sites/startswithabang/2018/11/17/ask-ethan-are-quantum-fields-real/ An illustration of the empty space of the Universe as consisting of quantum foam, where quantum... [+] NASA/CXC/M.WEISS
The Universe we perceive and view, all around us, isn't representative of what actually exists at a fundamental level. Instead of continuous, solid objects, matter is composed of indivisible quantum particles, held together through invisible forces that act across empty space. Both the particles themselves and the forces can be described by an underlying structure: quantum fields, which describe everything we know about all the particles and antiparticles of the Standard Model. But are these quantum fields real? And just what do they tell us? That's what Aaron Weiss wants to know, as he asks: I would be very interested in a post about quantum fields. Are they generally/universally believed to be real and the most fundamental aspect of our universe or just a mathematical construct? I've read that there are 24 fundamental quantum fields: 12 fields for fermions and 12 for bosons. But I've also read about quantum fields for atoms, molecules, etc. How does that work? Does everything emerge from these 24 fields and their interactions? Let's start with what a quantum field actually is. The proton's structure, modeled along with its attendant fields, show how even though it's made out... [+] BROOKHAVEN NATIONAL LABORATORY PROMOTED In physics, a field, in general, describes what some property of the Universe is everywhere in space. It has to have a magnitude: an amount that the field is present. It may or may not have a direction associated with it; some fields do, like electric fields, some don't, like voltage fields. When all we had were classical fields, we stated that the fields must have some kind of source, like particles, which results in the fields existing all throughout space.
In quantum physics, though, this seemingly self-evident fact is no longer true. Whereas classical physics defines quantities like position and momentum as properties of a particle, and those properties would generate a corresponding field, quantum physics treats them differently. Instead of quantities, position and momentum (among other quantities) now become operators, which allow us to derive all the quantum weirdness you've heard so much about. Through a herculean effort of the part of theoretical physicists, the muon magnetic moment has been... [+] 2012 AMERICAN PHYSICAL SOCIETY A quantity like an electron no longer has a well-defined position or momentum, but rather a wavefunction that describes the probability distribution of all possible positions and momenta. You may have heard these words before, but have you ever thought about what that actually means? It means that the electron isn't a particle at all. It's not something you can put your finger on and declare, "the electron is here, moving with this particular speed in this particular direction." You can only state what the overall properties are, on average, of the space in which the electron exists. This diagram illustrates the inherent uncertainty relation between position and momentum. When one... [+] WIKIMEDIA COMMONS USER MASCHEN
That doesn't sound very particle-like, does it? In fact, that sounds more field-like: some property of the Universe everywhere in space. That's because, in quantum field theory (QFT), quantum fields aren't generated by matter. Instead, what we interpret as "matter" is itself a quantum field.
And these quantum fields, themselves, are made up of particles.
The electromagnetic field? Made of particles called photons.
The strong nuclear field, that holds protons and neutrons together? Made up of particles called gluons.
The weak nuclear field, responsible for radioactive decays? Made of particles called W-and-Z bosons.
Even the gravitational field, if we try and formulate a quantum version of gravity? Made of particles called gravitons.
Yes, even the gravitational waves that LIGO detected, as smooth and continuous as they appeared, should be made of individual quantum particles. Gravitational waves propagate in one direction, alternately expanding and compressing space in... [+] M. PÖSSEL/EINSTEIN ONLINE
The reason we can use these terms of particles and fields interchangeably, in QFT, is because the quantum fields themselves encode all the information for everything. Have a particle and antiparticle annihilating? That's described by equal-and-opposite excitations of a quantum field. Want to describe the spontaneous creation of particle-antiparticle pairs of particles? That's also due to excitations of a quantum field. A visualization of QCD illustrates how particle/antiparticle pairs pop out of the quantum vacuum for... [+] DEREK B. LEINWEBER
Even particles themselves, like electrons, are just excited states of a quantum field. Every particle in the Universe, as we understand it, is a ripple, or excitation, or bundle-of-energy, of the underlying quantum field. This is true for the quarks, the gluons, the Higgs boson, and for all the other particles of the Standard Model. The Standard Model of particle physics accounts for three of the four forces (excepting gravity),... [+] CONTEMPORARY PHYSICS EDUCATION PROJECT / DOE / NSF / LBNL
So how many fundamental quantum fields are there? Well, that depends on how you look at the theory. In the simplest QFT that describes our reality, the quantum electrodynamics of Julian Schwinger, Shinichiro Tomonaga and Richard Feynman, there are only two quantum fields: the electromagnetic field and the electron field. They interact; they transfer energy and momentum and angular momentum; excitations are created and destroyed. Every excitation that's possible has a reverse excitation that's also possible, which is why this theory implies the existence of positrons (antimatter counterparts of electrons). In addition, photons exist, too, as the particle equivalents of the electromagnetic field. When we take all the forces that we understand, i.e., not including gravity, and write down the QFT version of them, we arrive at the predictions of the Standard Model. The particles and antiparticles of the Standard Model have now all been directly detected, with the... [+] E. SIEGEL / BEYOND THE GALAXY
This is where the idea of 12 fermion fields and 12 boson fields come from. These fields are excitations of the underlying theories (the Standard Model) that describe the known Universe in its entirety, and include:
The six (up, down, strange, charm, bottom, top) quarks, and their antiquark counterparts,
The three charged (electron, muon, tau) and three neutral (electron neutrino, muon neutrino, tau neutrino) leptons, and their antimatter counterparts,
The eight gluons (because of the eight possible color combinations),
The two weak (W-and-Z) bosons,
The one electromagnetic (photon) boson,
And the Higgs boson.
The quarks and leptons are fermions, which is why they have antimatter counterparts, and the W boson comes in two equal-and-opposite varieties (positively and negatively charged), but all told, there are 24 unique, fundamental excitations of quantum fields possible. This is where the "24 fields" idea comes from. Hydrogen density plots for an electron in a variety of quantum states. While three quantum numbers... [+] POORLENO / WIKIMEDIA COMMONS
So what about complex systems, then, like protons, atoms, molecules, and more? You have to understand that just as the 24 fields are actually excitations of the underlying QFT that describes our physical reality, these complex systems are more than just combinations of these fields put together into some stable or quasi-stable bound state. Instead, it's more accurate to view the entire Universe as a complicated quantum field that, itself, contains all of physics. Quantum fields can describe an arbitrarily large number of particles that interact in all ways our theories can conceivably allow. And they do this not in some vacuum of empty space, but amidst a background of not-so-empty-space, which plays by the rules of QFT, too. Visualization of a quantum field theory calculation showing virtual particles in the quantum vacuum.... [+] DEREK LEINWEBER
Particles, antiparticles, and all sorts of excitations of the fields are constantly being created-and-destroyed. Reality is fundamentally different from our classical picture of a smooth, continuous, well-defined Universe. Although it's true that these quantum fields began as a mathematical construct, they describe our physical, observable reality more accurately than any other theory we've concocted. They allow us to make incredibly precise predictions about what the results of any experiment involving the quanta of the Standard Model will yield: predictions that have been borne out by every experiment sensitive enough to test them.
The Universe may not be an intuitive place, but as far as any physical theory can call itself reflective of reality, QFT has no equal in terms of its power. As long as physics remains an experimental science, this will be the standard that any candidate theory will have to supersede.
__________________________________________________________________
Ask Ethan: How Do Quantum Fields Create Particles?
Ethan Siegel Senior Contributor Starts With A Bang Contributor Group Science The Universe is out there, waiting for you to discover it.
https://www.forbes.com/sites/startswithabang/2019/01/13/ask-ethan-how-do-quantum-fields-create-particles/?sh=2082afe6330a
At the high temperatures achieved in the very young Universe, not only can particles and photons be... [+] BROOKHAVEN NATIONAL LABORATORY
What is our Universe made out of? At a fundamental level, to the best of our knowledge, the answer is simple: particles and fields. The type of matter that makes up humans, Earth, and all the stars, for example, is all composed of the known particles of the Standard Model. Dark matter is theorized to be a particle, while dark energy is theorized to be a field inherent to space itself. But all the particles that exist, at the core of their nature, are just excited quantum fields themselves. What gives them the properties that they have? That's the topic of this week's question, coming to us from Richard Hunt, who wants to know:
I have a question about Quantum fields. If we model particle properties as excitations of various independent fields (Higgs field for mass, EM field for charge etc) then what causes these excitation waves to travel around together? Is there really some kind of particle entity underlying these waves?
In other words: what makes a particle have the properties that it does? Let's take a deep look.
The particles and antiparticles of the Standard Model have now all been directly detected, with the... [+]
E. SIEGEL / BEYOND THE GALAXY
The particles that we know of have traits that appear to be inherent to them. All particles of the same type — electrons, muons, up quarks, Z-bosons, etc. — are, at some level, indistinguishable from one another. They all have a slew of properties that all other particles of the same type share, including:
mass,
electric charge,
weak hypercharge,
spin (inherent angular momentum),
color charge,
baryon number,
lepton number,
lepton family number,
and more. Some particles have a value of zero for many of these quantities; others have non-zero values for almost all of them. But somehow, every particle that exists contains all of these particular, intrinsic properties bound together in a single, stable, "quantum state" we call a particular particle. The rest masses of the fundamental particles in the Universe determine when and under what... [+] FIG. 15-04A FROM UNIVERSE-REVIEW.CA
Underlying all of it, there are a variety of fields that exist in the Universe. There's the Higgs field, for example, which is a quantum field that permeates all of space. The Higgs is a relatively simple example of a field, even though the particle that arose from its behavior — the Higgs boson — was the last one ever to be discovered. The electromagnetic (QED) field and color-charge (QCD) field, among others, are also fundamental quantum fields.
Here's how it works: the field exists everywhere in space, even when there are no particles present. The field is quantum in nature, which means it has a lowest-energy state that we call the zero-point energy, whose value may or may not be zero. Across different locations in space and time, the value of the field fluctuates, just like all quantum fields do. The quantum Universe, to the best of our understanding, has rules governing its fundamental indeterminism. Visualization of a quantum field theory calculation showing virtual particles in the quantum vacuum.... [+] DEREK LEINWEBER
So if everything is fields, then what is a particle? You may have heard a phrase before: that particles are excitations of quantum fields. In other words, these are quantum fields not in their lowest-energy — or zero-point — state, but in some higher-energy state. But exactly how this works is a bit tricky. Up until this point, we've been thinking of fields in terms of empty space: the quantum fields we're discussing exist everywhere. But particles don't exist everywhere at once. On the contrary, they're what we call localized, or confined to a particular region of space. The simplest way to visualize this is to impose some sort of boundary conditions: some region of space that can be different from purely empty space. Trajectories of a particle in a box (also called an infinite square well) in classical mechanics (A)... [+] STEVE BYRNES / SBYRNES321 OF WIKIMEDIA COMMONS
In our pre-quantum picture of the Universe, particles are simply points and nothing more: individual entities with a set of properties assigned to them. But we know that in the quantum Universe, we have to replace particles with wavefunctions, which are a probabilistic set of parameters that replace classical quantities like "position" or "momentum." Instead of unique values, there are a set of possible values that a quantum field can take on. Some of the properties associated with a particle are continuous, like position, while others are discrete. The discrete ones are the most interesting in terms of fundamental particle properties, since those can only take on specific values that are defined by the characteristic conditions that the Universe sets out. A guitar string, on its own, can vibrate in an infinite number of vibrational modes, corresponding... [+] GETTY
A simple way to visualize this is to imagine a guitar. On a guitar, you have six strings of different thicknesses, where we can view thickness as a fundamental property of the string. If all you had were these strings (and no guitar), and you asked the question of the number of different possible ways these strings could vibrate, you'd wind up with an infinite number of allowable outcomes.
But guitars don't offer an infinite set of possibilities at all. We have boundary conditions on those strings:
the effective length of each string is constrained by the start-and-end points,
the number of possible excitations are constrained by the positions of the frets on the fretboard,
the vibrational modes are constrained by geometry and the music of overtones,
and the possible sounds it can make are constrained by the tension of each string.
These properties are uniquely determined by the size, string properties, and tuning of each individual guitar. The Standard Model Lagrangian is a single equation encapsulating the particles and interactions of... [+] THOMAS GUTIERREZ, WHO INSISTS THERE IS ONE 'SIGN ERROR' IN THIS EQUATION
In the case of our Standard Model particles, there are also a finite set of possibilities. They arise from a specific type of quantum field theory: a gauge theory. Gauge theories are invariant under a slew of transformations (like speed boosts, position translations, etc.) that our physical laws should also be invariant under.
The Standard Model in particular comes from a quantum field theory made up of three groups (as in the mathematics of Lie groups) all tied together:
SU(3), a group that's made of 3 × 3 matrices, which describes the strong interaction,
SU(2), a group that's made of 2 × 2 matrices, which describes the weak interaction,
and U(1), known as the circle group and made of all complex numbers with an absolute value of 1, which describes the electromagnetic interaction.
Put these all together in the proper way — SU(3) × SU(2) × U(1) — and you get our Standard Model. This diagram displays the structure of the standard model (in a way that displays the key... [+] LATHAM BOYLE AND MARDUS OF WIKIMEDIA COMMONS
The Standard Model isn't just a set of laws of physics, but provides proverbial boundary conditions that describe the spectrum of particles that can exist. Because the Standard Model isn't just made of a single quantum field in isolation, but all of the fundamental ones (except gravity) working together, the spectrum of particles we wind up with has a fixed set of properties.
This is determined by the specific mathematical structure — SU(3) × SU(2) × U(1) — that underlies the Standard Model. Each particle corresponds to the fundamental quantum fields of the Universe all excited in a particular way, with explicit couplings to the full suite of fields. This determines their particle properties, like:
mass,
electric charge,
color charge,
weak hypercharge,
lepton number,
baryon number,
lepton family number,
and spin.
The pattern of weak isospin, T_3, and weak hypercharge, Y_W, and color charge of all known... [+] CJEAN42 OF WIKIMEDIA COMMONS
If the Standard Model were all there were, no other combinations would be allowed. The Standard Model gives you fermion fields, which correspond to the matter particles (quarks and leptons), as well as boson fields, which correspond to the force-carrying particles (gluons, weak bosons, and photon), as well as the Higgs.
The Standard Model was built with a set of symmetries in mind, and the particular ways these symmetries break determine the spectrum of allowed particles. They still require us to put in the fundamental constants that determine the specific values of particle properties, but the generic properties of a theory with:
6 quarks and antiquarks with three colors each,
3 charged leptons and antileptons,
3 neutrinos and antineutrinos,
8 massless gluons,
3 weak bosons,
1 massless photon,
and 1 Higgs boson,
are determined by the Standard Model itself. The Standard Model of particle physics accounts for three of the four forces (excepting gravity),... [+] CONTEMPORARY PHYSICS EDUCATION PROJECT / DOE / NSF / LBNL
So how do we get quantum particles with the properties we do? Three things come together:
1. We have the laws of quantum field theory, which describe the fields permeating all of space that can be excited to different characteristic states.
2. We have the mathematical structure of the Standard Model, which dictates the allowable combinations of fields and their configurations (i.e., particles) that can exist.
3. We have the fundamental constants, which provide the values of specific properties to each allowable combination: the properties of each particle.
And there may be more. The Standard Model may describe reality extremely well, but it doesn't include everything. It doesn't account for dark matter. Or dark energy. Or the origin of the matter-antimatter asymmetry. Or the reasons behind the values of our fundamental constants.
The Standard Model only provides the allowable configurations we know of. If neutrinos and dark matter are any indication, there ought to be more. One of the prime goals of 21st century science is to find out what else is there. Welcome to the cutting-edge frontier of modern physics.
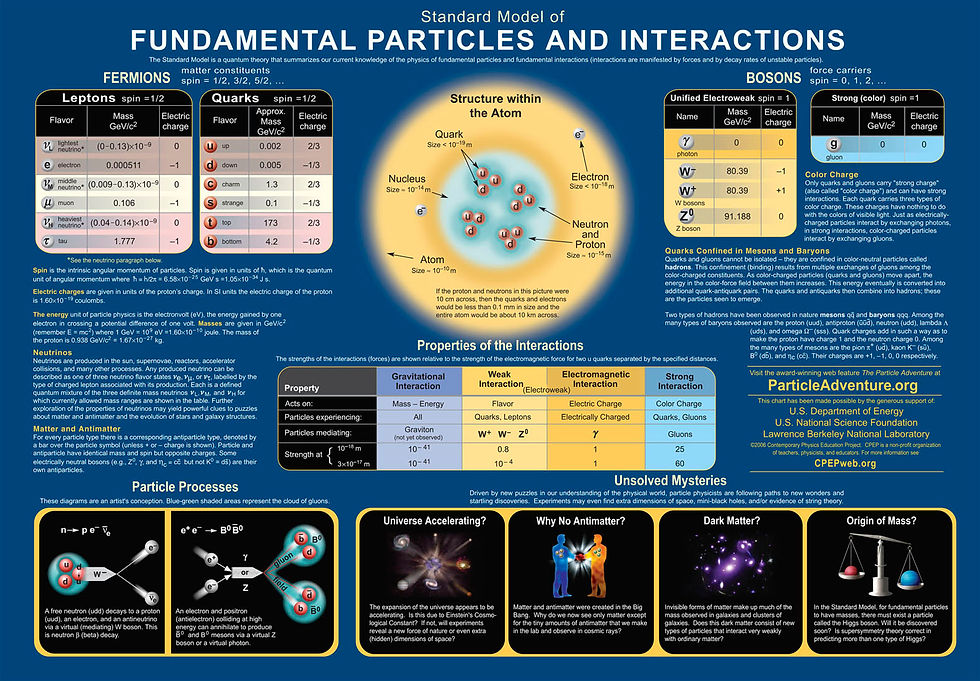
__________________________________________________________________________
What is a Force? Revisited.
Fields Viewpoint:
(Everyday Forces)
A Force arises from the action of the field of one particle upon another particle, and vice versa. Traditional View.
Everyday Contact Forces. And, Non-Contact Forces. Action at a Distance.
A 'Force' Involves "The Transfer Of Momentum".
Force-Carrier Particles Viewpoint:
(In Subatomic Particle Interactions)
Or, A 'Force' arises from a force-carrier particle exchanging between from one matter particle to another matter particle. The force-carrier particle mediates "The Transfer of Momentum". The force-carrier exchange manifests as / gives rise to / results in the action of one matter particle upon another matter particle. Force-carrier particles may be real particles or virtual particles.
How Does It Work? A Force-Carrier Analogy. How A Repulsive Force Manifests / Arises? Two basketball players on ice-skates or each in his own row boat on water. The basketball players are the particles here. The basketball one throws to the other is the force-carrier here. For a Repulsive Force, one player throws the ball to the other. He moves back. The other player catches/accepts the ball. A transfer of momentum. The other player moves back also. Both move away from each other as if by some repulsive force (created by the force-carrier exchange).
How Does An Attractive Force Manifests / Arises Then? How does that work in this analogy? One player throws the ball with an elastic cord attached to the ball and the other cord end to him. The other player catches/accepts the ball. Both are pulled toward each other by the elastic cord. Or maybe, one player throws a bommerang in the opposite direction away from the other player. He moves toward the other player. The other player catches/accepts the returning bommerang toward them. A transfer of momentum. He moves toward the other player too. Both move toward each other as if by some attractive force (created by the force-carrier exchange).
Indeed, a force-carrier exchange between matter particles does create either a repulsive force or an attractive force between the matter particles. That's the effect of force-carrier particles on matter particles. The Force manifests / arises / results from the force-carriers. Force-Carriers ------> Forces.
Subatomic Particle Interactions involve the exchange of fundamental force-carriers between matter particles. So, we can think of each Fundamental Force-Carrier Particle giving rise to their corresponding Fundamental Force/Interaction.
Fundamental Forces Are Not Like Everyday Forces With A Direct Nature.
Instead, Fundamental Forces Results InDirectly From Their Fundamental Force-Carrier Particle Being Exchanged Between Subatomic Matter Particles.
There are Four Fundamental Forces Corresponding To The Four Fundamental Force-Carrier Particles. But at the Subatomic Level, it is best to keep in mind and think of Fundamental Interactions rather than as Fundamental Forces.
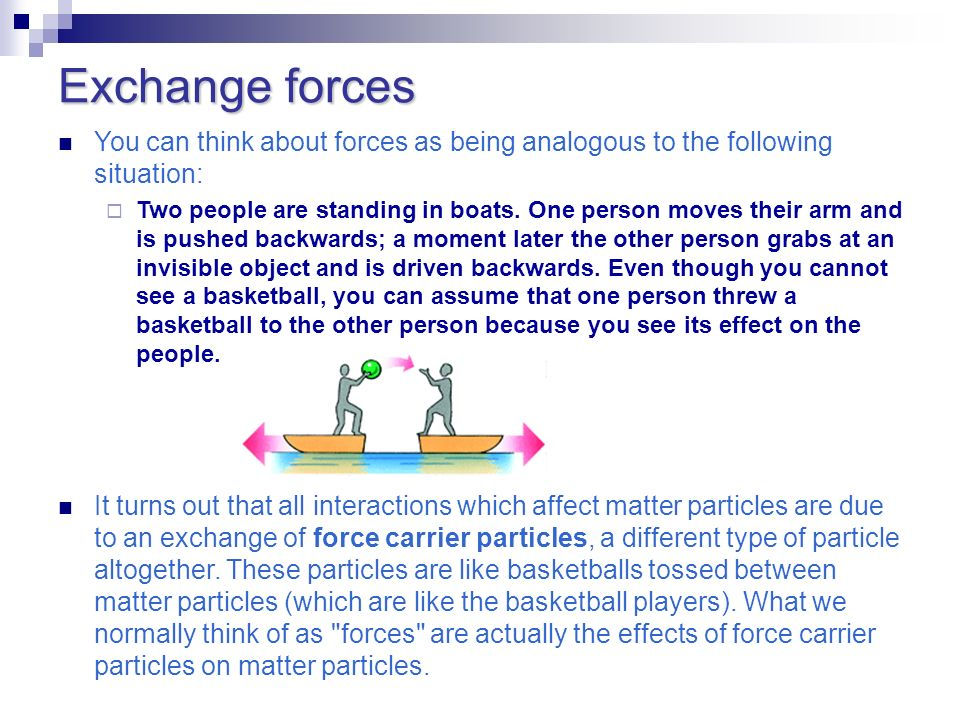
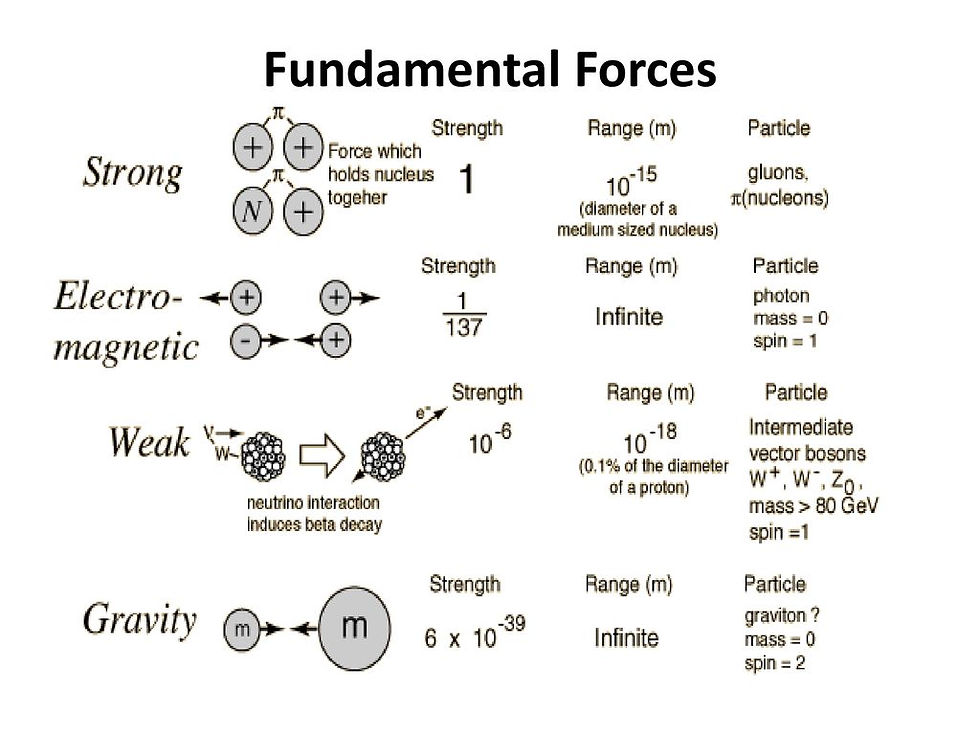
_______________________________________________________________
Ask Ethan: When Did The Universe Get Its First Quantum Fields?
(Spoiler: Since The Inflation Period. Of The Big Bang.)
Ethan Siegel Senior Contributor Starts With A Bang Contributor Group Science The Universe is out there, waiting for you to discover it.
https://www.forbes.com/sites/startswithabang/2020/10/09/ask-ethan-when-did-the-universe-get-its-first-quantum-fields/

Quantum gravity tries to combine Einstein’s general theory of relativity with quantum mechanics. ... [+] SLAC NATIONAL ACCELERATOR LABORATORY
No matter how we look at the Universe — at low temperatures or ultra-high energies, from our own backyard to the most distant recesses of the observable cosmos — we find that the same laws of physics apply. The fundamental constants remain the same; gravitation appears to behave the same; the quantum transitions and relativistic effects are identical. At all points in time, at least for the parts of the Universe we can observe, General Relativity (governing gravity) and Quantum Field Theory (governing the other known forces) appear to apply in the exact same form we find them appearing here on Earth. But has it always been this way? Is there a time where the Universe didn’t have the same quantum fields in it, or perhaps no quantum fields at all? That’s what Chris Shaw wants to know, asking: “When did the first quantum fields form in the universe? Have they been there since the Big Bang or even from the inflationary period before?” Perhaps surprisingly, quantum fields are there even under conditions where you might not expect them. Here’s what we know so far. Magnetic field lines, as illustrated by a bar magnet: a magnetic dipole, with a north and south pole ... [+] NEWTON HENRY BLACK, HARVEY N. DAVIS (1913) PRACTICAL PHYSICSPROMOTED When we think about fields, most of us conceive of them the same way scientists did back in the 1800s: when you have some type of source — like an electric charge or a permanent magnet — it creates a field around it at every point in space. That field exists whether or not there are other particles there to be affected by it, but you can detect the presence of that field (as well as what it affects and how) by observing what happens to charges of various types that interact with that field. Iron filings, which themselves can get magnetized, respond to magnetic fields by aligning along the direction of a field. Electric charges, in the presence of an electric field (or in motion in the presence of a magnetic field), will experience a force that accelerates them dependent on the strength of the field.
Even gravitation, whether in Einstein’s or Newton’s conception, can be visualized as a field: where matter or energy of any form will respond to the cumulative gravitational effects at its location in space, determining its future trajectory. The gravitational field, or the gravitational influence of all objects combined on a region of space ... [+]NASA
This visualization, as useful and common as it might be, only works in non-quantum settings, however. It’s an excellent illustration of how classical fields work, but we live in a fundamentally quantum reality. What we conceive of in the classical world — that fields are smooth, continuous, and that its properties can exist anywhere along a spectrum from a theoretical minimum to a theoretical maximum — no longer applies in a quantum Universe.
Instead, a quantum field isn’t only present where you have a source (like a mass or a charge), but rather is omnipresent: everywhere. If you have charges present, such as:
masses (for gravity),
electric charges (for electromagnetism),
a particle with a nonzero weak hypercharge (for the weak nuclear force),
or a color charge (for the strong nuclear force),
they behave like an excited state of the field, but the field is present regardless of the presence or absence of charges. What’s more: the field is quantized, and its zero-point energy, or the lowest energy level it can occupy, is allowed to have non-zero values. Today, Feynman diagrams are used in calculating every fundamental interaction spanning the strong, ... [+] DE CARVALHO, VANUILDO S. ET AL. NUCL.PHYS. B875 (2013) 738-756
In other words, “empty space” as we understand it, with no charges, masses, or other sources of the field in it, isn’t exactly empty, but still has these quantum fields present within it. That means that quantum fluctuations, which arise as a consequence of the quantum nature of these fields combined with Heisenberg’s uncertainty principle, exist throughout all of space as well, occupying every possible quantum mode and state (with specific, and in-principle computable, probabilities for those states to be occupied) allowable by the system. You might be skeptical of this approach, thinking to yourself something along the lines of, “well, so what? Quantum field theory is just one approach to doing calculations; it’s not like there’s an experimental test of whether these quantum fields exist in the reality of empty space or not.” But there is a test. You can take two parallel, conducting plates, and place them in the most perfect vacuum you can create: where there’s no matter and no sources of any type, just the quantum fields inherent to empty space, including the fundamental quantum electromagnetic field. An illustration of the Casimir effect, and how the forces (and allowed/forbidden states of the ... [+] EMOK / WIKIMEDIA COMMONS
Outside of those two plates, all of the possible states of these quantum fields are allowed, as there are no restrictions on which modes are forbidden. But inside those plates, only a subset of those quantum fields are allowed, as there are boundary conditions that prevent certain electromagnetic waves — and hence, certain excitations of the quantum fields — from existing. Even without any sources for those electromagnetic waves, those excited field states (or, if it makes it easier for you to visualize it, those virtual modes and particles) are different inside and outside those plates, creating a net force on those plates: the Casimir force.
Predicted by scientist Hendrik Casimir back in 1948, the first experimental detection of this force didn’t occur until 1997, when physicist Steve Lamoreaux accomplished the feat and got a result that was within 5% of Casimir’s predicted value for the system. These quantum fields really do exist all throughout space, and experiments not only show that they do exist, but show us the magnitude of their effects as well. The contributions of the known quantum fields to the vacuum cannot be practically calculated today, ... [+] DEREK LEINWEBER
One of the concepts that physicists wonder about is whether the quantum fields we know about — the ones that are a part of the Standard Model plus whatever the (assumed) quantum fields associated with gravity are — make up all of the quantum fields permeating empty space, or whether there are others. For example, could there be additional quantum fields arising from:
whatever is responsible for dark matter,
whatever phenomenon or field causes dark energy,
any fields left over from the Universe’s inflationary phase,
new fields or interactions arising from some sort of grand unification,
or any other exotic new physics (including, but not limited to, new forces or particles) that may exist beyond the Standard Model.
Although the laws of physics don’t change over the conditions we’ve observed them, from here to particle accelerators to the earliest observable stages of the Big Bang, the properties of quantum fields ensure that the strength of quantum couplings, corresponding to the forces experienced by particles owing to these quantum fields, actually change as a function of energy and temperature. When you view the coupling constants as a function of energy on a log-log scale, they appear to ... [+] CERN (EUROPEAN ORGANIZATION FOR NUCLEAR RESEARCH), 2001
In physics, we call this “the running of the coupling constants,” and you can visualize it as having more excited-state modes occupied by these virtual quantum particles as compared to greater fractions of lower-energy, ground-state modes. Although this doesn’t imply that the quantum fields that govern the Universe were different at earlier, higher-energy times, it is suggestive of something: that perhaps these coupling constants unify at some point, indicating that the strong, weak, and electromagnetic forces might have arisen from a grander theory where all the forces are unified. That framework not only offers up the possibility that additional quantum fields might show up and reveal their effects at those high energies, but that there might be some sort of “ultimate unification,” or a theory of everything. If such a state exists, you can imagine that as the ultimate version of a restored symmetry: like putting a ball at the absolute top of the highest mountain peak on a planet. When a symmetry is restored (yellow ball at the top), everything is symmetric, and there is no ... [+] PHYS. TODAY 66, 12, 28 (2013)
When a symmetry breaks, that’s like rolling down the hill and into the lowest point of whatever valley it happens to fall into. But if you bring the ball back up to the top of the hill multiple times and balance it as best as you can, it won’t necessarily roll down the same way every time. Depending on factors like:
very slight differences in initial conditions,
small, even quantum, fluctuations,
at what rate the Universe expands or cools,
and the presence or absence of novel field couplings,
that broken symmetry can wind up in one of any number of possible final states. There is no guarantee, if we ran the clock back to some extremely early time, the laws of physics and the fundamental constants that emerged to govern our Universe would be the same each and every time we did it. Just like we believe we “won” the cosmic lottery by having human life arise on Earth (and most probably nowhere else in the Universe), it’s possible we won the cosmic lottery by getting the laws and constants that we did. An illustration of multiple, independent Universes, causally disconnected from one another in an ... [+] OZYTIVE / PUBLIC DOMAIN
When we run the clock backwards to the earliest stages of the hot Big Bang, however, we see no evidence that the Universe ever reached sufficient temperatures that this theorized unification (and restoration of a symmetry) actually occurred. When you break a symmetry, particles are produced, and if this type of grand unification occurred, it should have produced a large number of magnetic monopoles: particles that clearly don’t exist in our Universe. If the quantum fields that we know of today emerged from some earlier state where they didn’t exist previously, that state must be confined to a realm before the hot Big Bang. Does that mean they could have been created during cosmic inflation? It’s possible, but we don’t know. Based on the inferred limits on the energies achieved during inflation — which themselves come from the fluctuations generated during inflation that are imprinted in our CMB and large-scale structure today — inflation might not have reached sufficient energies for this to occur. Although successful models of inflation demand a multiverse, it’s still speculative to presume that the constants or laws are different in different “pocket Universes.” The quantum fluctuations that occur during inflation do indeed get stretched across the Universe, ... [+] E. SIEGEL / BEYOND THE GALAXY
However, one thing that is certain is that quantum fields of some variety must have still existed during inflation. They may or may not be the same quantum fields that exist today, and there may have been additional quantum fields over and above the ones we know of and have today, but they had to exist. How do we know? Because the fluctuations that we see in the Universe, the ones that gave rise to the cosmic structure that eventually formed, match exactly the ones predicted to arise from fluctuating quantum fields that existed during inflation.
Those fluctuations, the ones that normally occur on tiny, microscopic quantum scales, get stretched across the Universe during inflation, get translated into temperature and density fluctuations at the start of the hot Big Bang, and imprint themselves irrevocably onto the Universe. The fact that we’ve observed these fluctuations and their consequences tell us, quite definitively, that those quantum fields did exist during inflation.
For as long as spacetime has existed, some version of quantum fields must have existed as well. But whatever occurred in our Universe prior to the final tiny fraction-of-a-second of inflation can never be observed or accessed from within our observable Universe. In the absence of evidence, we are bound to probe the limits of what’s known and match them up with whatever’s left as a possibility. However fun and instructive it may be to speculate, the truth is we simply don’t know.
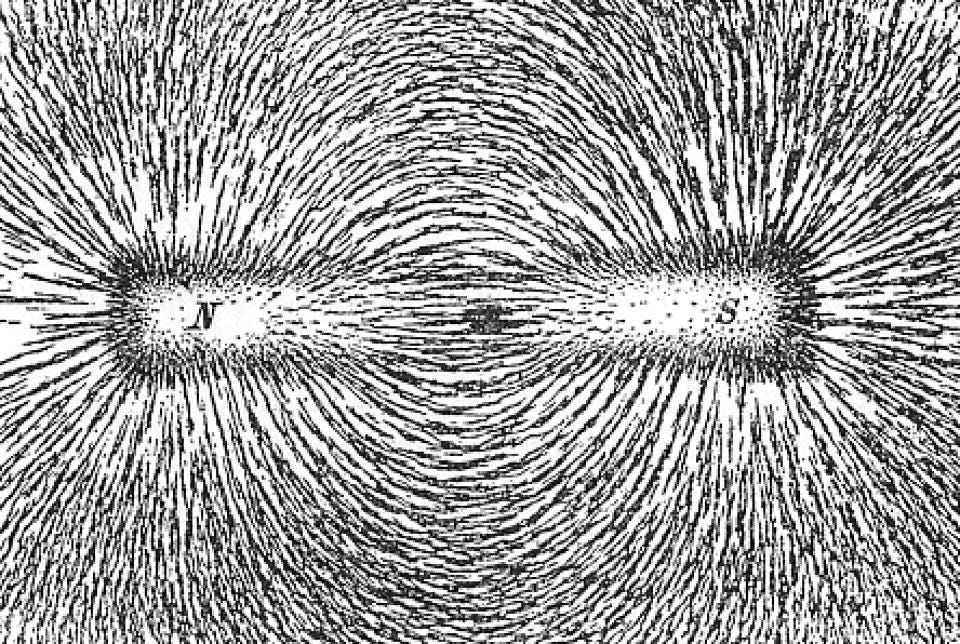


コメント